
Once nucleated at specific sites the epitaxial front can then proceed, the kinetics and mode of realignment depending on the density of nucleation sites and on the growth velocity. In the early stages of interface realignment the process is controlled by oxide clustering, arising from the evolution of the native oxide layer, and by the density of grain boundaries intersecting the interface with the single crystal. It is shown that these processes can be interpreted within an Avrami-Mehl-Johnson nucleation and growth scheme. These two different modes arise from the doping enhancement of the interface kinetics. For undoped layers a quasi planar interface motion occurs, while in highly doped polycrystalline layers the realignment proceeds via the formation of epitaxial columns and their lateral growth. Two different realignment modes are observed. The roles of the mucrystalline morphology, of the interfacial native oxide film, of the doping level and of the processing temperature on the realignment kinetics are addressed. In a 3-D crystal, such as a real one, each ball would also touch three on the plane above, and three on the plane below.Our recent work on the poly/single crystal Si interface evolution and on the epitaxial realignment under high temperature (∼ 1000☌) rapid thermal annealing is reviewed. Note that in this 2-D model, each ball touches six others. This pattern is typical of hexagonal close-packed and cubic close-packed lattices. In metals, close-packing of atoms is a very common structure. The balls within a grain arrange themselves into close-packed planes. The reason for this involves entropy: at all finite temperatures, there will be some disorder in the crystal. Note that diffusion occurs mainly near the top of the balls: those towards the middle and bottom do not easily move, as the photographs show.Įven in a single crystal, or large-grained sample, there are still vacancies, as the shot model shows. With great care, it may be possible to create a single crystal, as all the balls form a single pattern. Note the presence of vacancies in the structure. The following image sequence shows the behaviour of the shot model as it is rearranged by tapping, starting from a polycrystalline state with many small grains and ending with much larger grains. Occasionally, the "diffusion" process may cause two grains to join together, or for some grains to "grow".

This is similar to diffusion, in which case the tapping is analogous to thermal activation. Tapping of the model causes minor rearrangements of the balls, especially at the top of the "solid" region. In some places the balls form close-packed regions. One or two balls may be suspended above the main body by electrostatic forces: this is comparable to the vapour found above the crystal. The balls form a liquid-like structure.Īs the model is tilted towards the vertical, the balls pack closely together. When the shot model is held horizontally, so that the balls flow freely, the resulting structure is similar to a liquid. They tend to behave like the atoms in a crystal, and can show the same kind of defects. The model consists of many small ball bearings trapped in a single layer between two transparent plates. We can use a "shot model" to get a picture of crystal defects.

Crystal defects are important in determining many material properties, such as the rate of atomic diffusion and mechanical strength. Grain boundaries in polycrystals can be considered as two-dimensional defects in the perfect crystal lattice. The structure contains defects such as vacancies, where an atom is missing altogether, and dislocations, where the perfection of the structure is disrupted along a line.
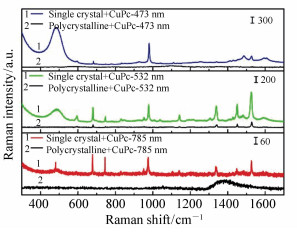
Within a single crystal or grain, the crystal structure is not perfect.
